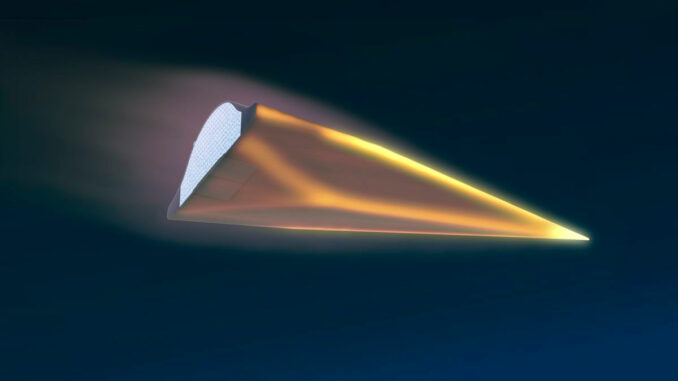
Hypersonic glide vehicles travel at Mach 5+ with atmospheric maneuverability. Explore design, speed, control, and strategic applications.
A hypersonic glide vehicle (HGV) is an advanced aerospace weapon system designed to travel at speeds exceeding Mach 5 while gliding through the atmosphere. Launched via rocket boosters, HGVs detach after the boost phase and re-enter the atmosphere to glide unpowered toward their targets. This phase allows for horizontal maneuvering, variable trajectories, and increased unpredictability. Unlike traditional ballistic missiles, HGVs can evade early detection and interception by altering their flight path mid-course. Typically flying at altitudes between 30 and 100 km, they exploit the aerodynamic lift and drag at the edge of space to sustain hypersonic speeds. Various nations have developed or tested operational HGVs, including China’s DF-ZF, Russia’s Avangard, and the U.S. DARPA Falcon project. The key engineering challenges involve thermal shielding, guidance control at hypersonic speeds, and material resistance. HGVs represent a critical shift in strategic deterrence and prompt global strike capabilities due to their high speed, low detectability, and maneuverability.
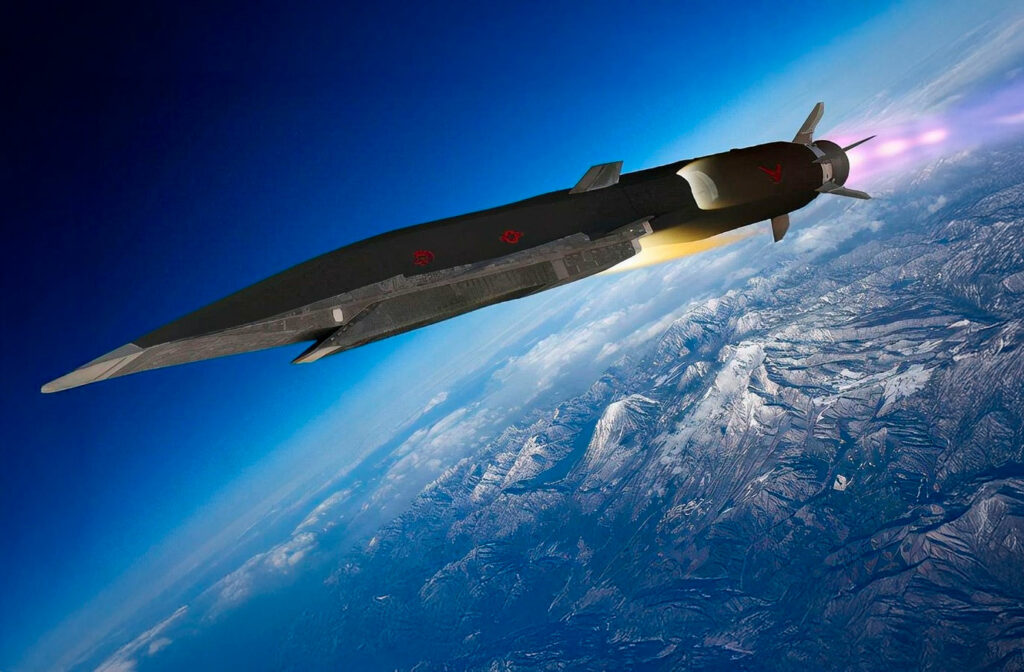
What is a hypersonic glide vehicle?
A hypersonic glide vehicle (HGV) is a type of unpowered, aerodynamic projectile capable of sustained atmospheric flight at speeds above Mach 5. Unlike traditional ballistic re-entry vehicles, HGVs use aerodynamic lift to glide long distances through the atmosphere after being launched by a booster rocket.
1. Launch and flight architecture
HGVs are not self-launching. They are typically mounted atop intercontinental ballistic missiles (ICBMs) or medium-range boosters. After launch, the vehicle follows a traditional boost phase. Upon reaching exo-atmospheric altitudes of ~100–150 km, the glide vehicle separates from the booster and re-enters the upper atmosphere.
The glide phase begins at altitudes between 30 and 100 km, where the vehicle uses the thin air for lift. Flight profiles vary significantly but usually remain within this mesospheric window to maintain maneuverability and thermal survivability. The final approach to the target is steep and fast, making interception difficult.
2. Aerodynamic control and maneuverability
Unlike ballistic vehicles with predictable paths, HGVs can execute lateral and vertical maneuvers during glide. These non-ballistic trajectories drastically reduce interception probability. Control surfaces and body shaping enable the vehicle to:
- Bank or pitch to change direction
- Perform s-turns or zig-zag patterns
- Adjust altitude dynamically
The lifting body design is crucial. Most HGVs feature blended wing-body shapes or waveriders to maximize lift-to-drag ratio while maintaining thermal stability. Key to maneuverability are active control surfaces, but at hypersonic speeds, aerodynamic forces and heating limit the material choices and actuation methods.
3. Materials and thermal protection
At speeds exceeding Mach 5, the vehicle encounters intense aerodynamic heating due to air compression and friction. Surface temperatures can reach 2,000°C or higher depending on altitude and velocity. HGVs require:
- Ultra-high-temperature ceramics (UHTCs) such as zirconium diboride (ZrB₂) or hafnium carbide (HfC)
- Carbon-carbon composites for leading edges
- Ablative coatings or thermal barrier layers for surface protection
The nose cone and leading surfaces bear the brunt of thermal load. Structural integrity at hypersonic temperatures is a primary engineering bottleneck. In some designs, passive insulation is supplemented with active cooling systems, such as transpiration cooling.
4. Guidance, navigation, and control (GNC)
Precision targeting at hypersonic speed is complex due to inertial errors, plasma interference, and short response windows. HGVs typically use:
- Inertial navigation systems (INS) for core trajectory tracking
- Star trackers or celestial navigation for mid-course correction
- GPS or GLONASS augmentation (if survivable in contested environments)
- Radar altimeters or optical seekers for terminal guidance
Due to high speeds, onboard processors must compute adaptive control commands in milliseconds, compensating for dynamic changes in pressure, temperature, and airflow.
5. Speed, altitude, and range characteristics
An operational HGV flies at Mach 5–20, depending on its launch system and glide path. Altitudes generally range from 30 km to 100 km in the glide phase. The range is a function of:
- Initial boost energy
- Vehicle lift-to-drag performance
- Atmospheric density and heating limits
For example:
- The Russian Avangard reportedly flies at Mach 20, with a range exceeding 6,000 km
- China’s DF-ZF operates in the Mach 5–10 range, with a glide phase of 1,500–2,000 km
- U.S. experimental systems, such as the HTV-2, achieved Mach 20 for ~4,000 km
The trade-off between maneuverability and thermal stress is critical: lower altitudes increase evasion but also raise heat loads.
6. Strategic and tactical implications
HGVs are part of prompt global strike (PGS) doctrine, allowing strikes within minutes to an hour globally. Their key advantages:
- Unpredictable trajectory, defeating radar tracking algorithms
- High speed, reducing reaction time
- Low radar cross-section (RCS) due to flattened body shapes and low-altitude flight
HGVs blur the line between strategic deterrence and conventional precision strike. A conventional HGV could neutralize hardened or mobile targets before countermeasures are deployed.
However, this unpredictability introduces instability in nuclear signaling, as adversaries may misinterpret a conventional HGV as a nuclear attack, triggering pre-emptive doctrines.
7. Known programs and development status
Russia:
- Avangard: Deployed since 2019; mounted on RS-28 Sarmat ICBM. Features maneuverable glide at Mach 20. Reportedly nuclear-armed.
China:
- DF-ZF: Operational since ~2020. Mounted on DF-17 MRBM. Estimated Mach 10, range up to 2,000 km. Believed to carry both nuclear and conventional warheads.
United States:
- DARPA Falcon HTV-2: Reached Mach 20, failed due to thermal disintegration.
- AGM-183A ARRW: Air-launched rapid response weapon under USAF. Failed multiple flight tests (as of 2023).
- OpFires and Glide Breaker: In development. Aimed at more survivable and controllable HGVs.
Others:
- India and France are exploring dual-capability HGVs.
- Australia, Japan, and the UK are investing in defensive counter-HGV systems.
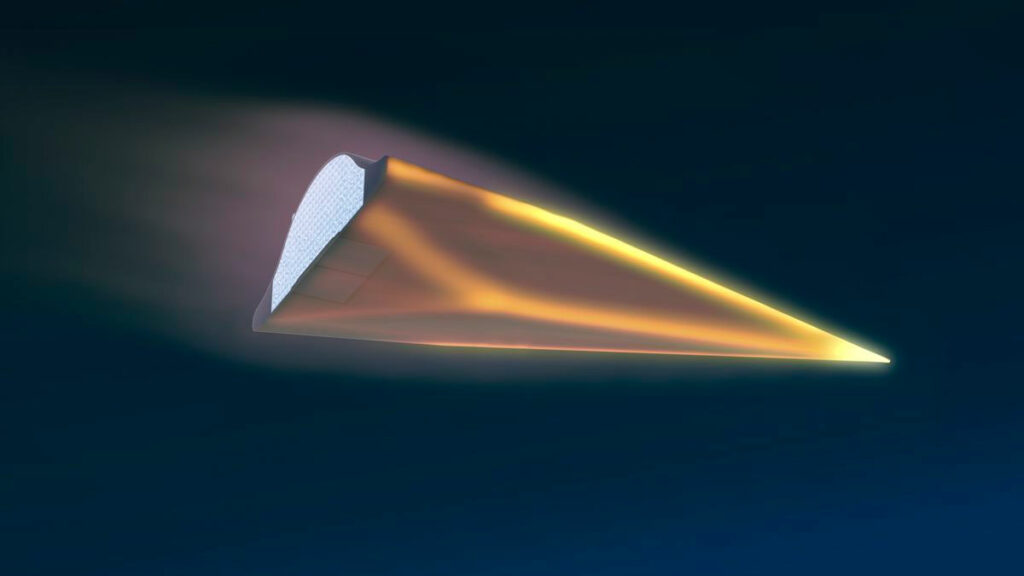
8. Interception challenges and countermeasures
Intercepting an HGV is significantly more difficult than engaging a ballistic missile. Reasons include:
- Non-predictable flight path
- Short sensor engagement windows
- High closing speeds (10–20 km/s)
Conventional missile defense systems such as THAAD or Aegis BMD are largely ineffective. Emerging solutions include:
- Directed energy weapons (DEWs)
- Hypersonic interceptors (e.g., Glide Phase Interceptor by MDA)
- Advanced radar using low-Earth orbit sensors to track low-flying HGVs
The development of machine-learning-based early warning systems is also underway to analyze flight patterns in real time.
A hypersonic glide vehicle merges rocket propulsion with aerodynamic control, enabling a unique class of high-speed, maneuverable weapons. Its defining characteristics—speed, trajectory flexibility, and atmospheric glide—make it a major advancement in strategic missile technology. Engineering challenges remain, particularly in thermal management and control at hypersonic speeds, but multiple nations are progressing rapidly. HGVs are reshaping the offensive-defensive balance in modern military doctrine, and their impact on global stability is under continuous scrutiny by defense planners worldwide.
War Wings Daily is an independant magazine.